1. Deep Tissue Imaging
Optical imaging in the deep brain is challenging because of tissue scattering. Scattered photons contribute to the background noise and degrade the signal-to-noise ratio (SNR) of fluorescent images. To tackle this challenge, my group developed several strategies, including active correction and post-processing algorithms, for imaging through scattering brain tissues.
2P-FOCUS: Two-Photon Microscopy with Active Scattering Correction
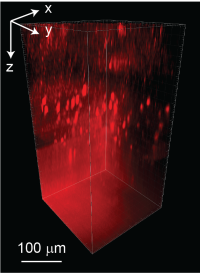
2P-FOCUS corrects scattering by utilizing the non-linearity of multiple-beam interference and two-photon excitation, eliminating the need for a guide star, iterative optimization, or measuring transmission or reflection matrices. It significantly enhanced two-photon fluorescence signals by several tens of folds when focusing through a bone sample, compared to cases without scattering compensation at equivalent excitation power. 2P-FOCUS also can correct scattering over large volumes by imaging neurons and cerebral blood vessels within a 230x230x500 µm3 volume in the mouse brain ex vivo.
Link: Zepeda, D., Li, Y. & Xue, Y*. Scattering compensation through Fourier-domain open-channel coupling in two-photon microscopy. arXiv [physics.optics] (2024)
HiLL: Two-Photon Microscopy with Structured Illumination
HiLL microscopy is based on line-scanning temporal focusing microscopy with structured illumination. It removes scattered photons with post-processing algorithms. It has been demonstrated with in vivo brain imaging.
Link: Xue, Y., Berry, K. P., Boivin, J. R., Wadduwage, D., Nedivi, E., & So, P. T. C. (2018). Scattering reduction by structured light illumination in line-scanning temporal focusing microscopy. Biomed. Opt. Express, BOE 9, 5654–5666 (2018)
MosTF: Two-Photon Microscopy with Photon Reassignment
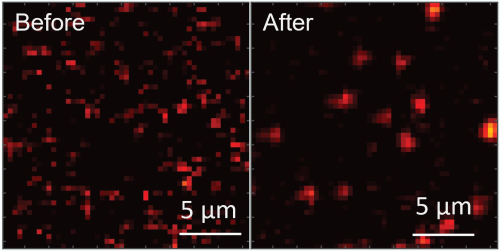
MosTF, referring to Multiline Orthogonal Scanning Temporal Focusing microscopy, captures a sequence of images at each scan location of the excitation line first, and then uses a reconstruction algorithm to reassign scattered photons back to their correct scan positions. MosTF has been demonstrated by acquiring neuronal images of mice in vivo and showed remarkable improvements in in vivo brain imaging.
Link: Xue, Y., Boivin, J. R., Wadduwage, D. N., Park, J. K., Nedivi, E., & So, P. T. C. (2024). Multiline orthogonal scanning temporal focusing (mosTF) microscopy for scattering reduction in in vivo brain imaging. Scientific Reports, 14(1), 10954.
Read more:
Wei, Z., Boivin J. R., Xue, Y., Chen, X., So, P. T. C., Nedivi, E., Wadduwage, D. N. 3D Deep Learning Enables Fast Imaging of Spines through Scattering Media by Temporal Focusing Microscopy. arXiv [eess.IV] (2019)
Zheng, C., Park, J. K., Yildirim, M., Boivin, J. R., Xue, Y., Sur, M., So, P. T. C., & Wadduwage, D. N. (2021). De-scattering with Excitation Patterning enables rapid wide-field imaging through scattering media. In Science Advances (Vol. 7, Issue 28, p. eaay5496). https://doi.org/10.1126/sciadv.aay5496
2. Multimodal Imaging
FDT: Fluorescence diffraction tomography using explicit neural fields
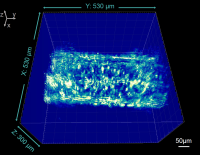
Simultaneous imaging of fluorescence-labeled and label-free phase objects in the same sample can provide distinct and complementary information. Unlike typical phase imaging with lasers or LEDs, we develop fluorescence diffraction tomography (FDT) with explicit neural fields to reconstruct the 3D RI of thick samples from diffracted fluorescence images captured on a defocused image plane. We successfully reconstructed the RI of 3D cultured label-free bovine myotubes in a 530 x 530 x 300 um3 volume using FDT.
Link: He, R., Li, Y., Chen, J., & Xue, Y. (2024). Fluorescence Diffraction Tomography using Explicit Neural Fields. In arXiv [physics.optics]. http://arxiv.org/abs/2407.16657
BRIEF: Measure fluorescence and refractive index together
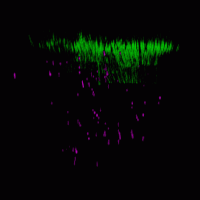
Conventional imaging techniques measure either labeled fluorescence or label-free RI of biological samples, but not both. We develop a bi-functional imaging method that reconstructs 3D fluorescence and refractive index from fluorescence images. Our method not only bridges fluorescence and RI imaging, but also can digitally correct multiple scattering in the fluorescence images by using the scattering potential recovered by the reconstructed 3D RI.
Link: Xue, Y., Ren, D. & Waller, L. Three-dimensional bi-functional refractive index and fluorescence microscopy (BRIEF). Biomed. Opt. Express (2022)
3. Holographic Optogenetic Stimulation and Imaging
3D-MAP: 3D Multi-site Random Access Photo-Stimulation and Imaging
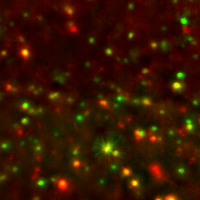
Optical control of neural ensemble activity is crucial for understanding brain function and disease, yet no technology can achieve optogenetic control of very large numbers of neurons at extremely fast rate over a large volume. We introduce a new one-photon light sculpting technique, that overcomes these limitations by modulating light dynamically, both in the spatial and in the angular domain at multi-kHz rates. 3D-MAP interrogates neural circuits in 3D and demonstrates simultaneous photostimulation and imaging of dozens of user-selected neurons in the intact mouse brain in vivo with high spatiotemporal resolution.
Link: Xue, Y., Waller, L., Adesnik, H*. & Pégard, N*. Three-dimensional multi-site random access photostimulation (3D-MAP). Elife 11, e73266 (2022)
saMMM: 3D scanless holographic imaging of neural activity
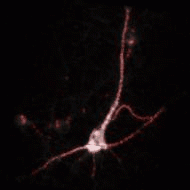
In conventional imaging systems, a 2D camera cannot record 3D information at a single shot, and fast imaging speed often leads to a compromise of image SNR. To improve system’s throughput without a penalty on SNR, we exploited computer-generated holography (CGH) to generate 3D holographic illumination and applied pupil encoding in the detection path to extend the depth of view and encode depth information with lateral patterns. This scanless imaging system reaches 100Hz volumetric imaging speed of 2.5 million voxels and will be a powerful tool to investigate the dynamic nature of neurons in the mouse brain.
Link: Xue, Y., Berry, K. P., Boivin, J. R., Rowlands, C. J., Takiguchi, Y., Nedivi, E., & So, P. T. C. Scanless volumetric imaging by selective access multifocal multiphoton microscopy. Optica vol. 6 76 (2019)
4. Super-Resolution Microscopy
SI-STED: STED Microscopy with 3D Structured Illumination for parallel scanning
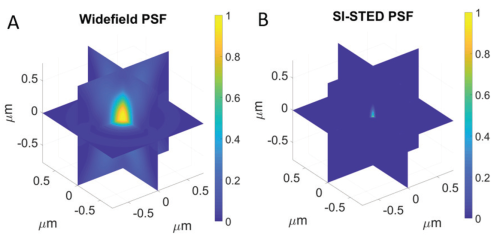
Stimulated emission depletion (STED) microscopy is typically a point-scanning method, limited its imaging speed. We develop the theoretical basis for extending STED microscopy to three dimensional imaging in parallel. This method uses structured illumination (SI) to generates a three dimensional depletion pattern. This method not only achieves axial super-resolution of STED microscopy but also greatly reduces photobleaching and photodamage for 3D volumetric imaging.
Link: Xue, Y. & So, P. T. C. Three-dimensional super-resolution high-throughput imaging by structured illumination STED microscopy. Opt. Express 26, 20920–20928 (2018)
Read more:
Xue, Y., Rowlands, C. J. & So, P. T. C. Parallel and flexible imaging using two-photon RESOLFT microscopy with spatial light modulator control. in Multiphoton Microscopy in the Biomedical Sciences XV vol. 9329 932927 (International Society for Optics and Photonics, 2015).
Gu, Z., Kuang, C., Hao, X., Xue, Y., Zheng, Z., and Liu, X. Methods for generating a dark spot using phase and polarization modulation light. Optik 124, 650–654 (2013).
Gu, Z., Kuang, C., Li, S., Xue, Y., Hao, X., Zheng, Z., and Liu, X. An interferential method for generating polarization-rotatable cylindrical vector beams. Opt. Commun. 286, 6–12 (2013).
Xue, Y., Kuang, C., Li, S., Gu, Z. & Liu, X. Sharper fluorescent super-resolution spot generated by azimuthally polarized beam in STED microscopy. Opt. Express 20, 17653–17666 (2012)
Ku, Y., Kuang, C., Hao, X., Xue, Y., Li, H., and Liu, X. Superenhanced three-dimensional confinement of light by compound metal-dielectric microspheres. Opt. Express, 20, 16981–16991 (2012).
Xue, Y., Kuang, C., Hao, X., Gu, Z., and Liu, X. A method for generating a three-dimensional dark spot using a radially polarized beam. J. Opt. 13, 125704 (2011).